Geology Anthology
by FCIT
Fossils, Rocks, and Time
by Lucy E. Edwards and John Pojeta, Jr.
Additional Information
- Year Published: 1997
- Language: English
- Country of Origin: United States of America
- Source: General Interest Publications of the U.S Geological Survey, (https://pubs.usgs.gov/gip, 1997)
-
Readability:
- Flesch–Kincaid Level: 10.9
- Word Count: 3,346
- Genre: Informational
- ✎ Cite This
Downloads
INTRODUCTION
A photographic collage depicting the diversity and evolution of life on Earth through the last 600 million years. The oldest fossils are at the bottom and youngest at the top. The size of each time interval is proportional to its duration.
We study our Earth for many reasons: to find water to drink or oil to run our cars or coal to heat our homes, to know where to expect earthquakes or landslides or floods, and to try to understand our natural surroundings. Earth is constantly changing—nothing on its surface is truly permanent. Rocks that are now on top of a mountain may once have been at the bottom of the sea. Thus, to understand the world we live on, we must add the dimension of time. We must study Earth’s history. When we talk about recorded history, time is measured in years, centuries, and tens of centuries. When we talk about Earth history, time is measured in millions and billions of years. Time is an everyday part of our lives. We keep track of time with a marvelous invention, the calendar, which is based on the movements of Earth in space. One spin of Earth on its axis is a day, and one trip around the Sun is a year. The modern calendar is a great achievement, developed over many thousands of years as theory and technology improved.
People who study Earth’s history also use a type of calendar, called the geologic time scale. It looks very different from the familiar calendar. In some ways, it is more like a book, and the rocks are its pages. Some of the pages are torn or missing, and the pages are not numbered, but geology gives us the tools to help us read this book.
PUTTING EVENTS IN ORDER
Scientists who study the past try to put events in their proper order. When we discuss events that happened in historical times, we often use dates or numbers, but we do not have to do so. Consider six historical events: the Wright brothers’ flight, the bicentennial of American independence, the First and Second World Wars, the first astronaut landing on the moon, and when television became common in homes. First, let’s try to put these events in order. Our knowledge of the words first and second tells us that the First World War came before the Second World War. We may know or may have been told that the landing of Neil Armstrong on the moon was seen by many people on television, but there was no television around when the Wright brothers flew at Kitty Hawk. Thus, we can order these three events: first Wright brothers’ flight, then television common in homes, then the landing on the moon. By a process of gathering evidence and making comparisons, we can eventually put all six events in the complete proper order: Wright brothers’ flight, First World War, Second World War, television common in homes, landing on the moon, and American bicentennial.
Because we have written records of the time each of these events happened, we can also put them in order by using numbers. The Wright brothers’ flight occurred in 1903, the First World War lasted from 1914 to 1918, and the Second World War lasted from 1939 to 1945. Televisions became part of our homes in the 1950’s, Neil Armstrong walked on the moon in 1969, and America celebrated 200 years of independence in 1976.
Written records are available for only a tiny fraction of the history of Earth. Understanding the rest of the history requires detective work: gathering the evidence and making comparisons.
Figures 3 and 4. The box at the left shows six events that occurred during the twentieth century. The bottom shows these events in relative order and numeric order.
THE RELATIVE TIME SCALE
Figure 5. The relative geologic time scale. The oldest time interval is at the bottom and the youngest is at the top.
Long before geologists had the means to recognize and express time in numbers of years before the present, they developed the geologic time scale. This time scale was developed gradually, mostly in Europe, over the eighteenth and nineteenth centuries. Earth’s history is subdivided into eons, which are subdivided into eras, which are subdivided into periods, which are subdivided into epochs. The names of these subdivisions, like Paleozoic or Cenozoic, may look daunting, but to the geologist there are clues in some of the words. For example, zoic refers to animal life, and paleo means ancient, meso means middle, and ceno means recent. So the relative order of the three youngest eras, first Paleoozoic, then Mesozoic, then Cenoozoic, is straightforward.
Fossils are the recognizable remains, such as bones, shells, or leaves, or other evidence, such as tracks, burrows, or impressions, of past life on Earth. Scientists who study fossils are called paleontologists. Remember that paleo means ancient; so a paleontologist studies ancient forms of life. Fossils are fundamental to the geologic time scale. The names of most of the eons and eras end in zoic, because these time intervals are often recognized on the basis of animal life. Rocks formed during the Proterozoic Eon may have fossils of relative simple organisms, such as bacteria, algae, and wormlike animals. Rocks formed during the Phanerozoic Eon may have fossils of complex animals and plants such as dinosaurs, mammals, and trees.
We study Earth’s history by studying the record of past events that is preserved in the rocks. The layers of the rocks are the pages in our history book. Most of the rocks exposed at the surface of Earth are sedimentary—formed from particles of older rocks that have been broken apart by water or wind. The gravel, sand, and mud settle to the bottom in rivers, lakes, and oceans. These sedimentary particles may bury living and dead animals and plants on the lake or sea bottom. With the passage of time and the accumulation of more particles, and often with chemical changes, the sediments at the bottom of the pile become rock. Gravel becomes a rock called conglomerate, sand becomes sandstone, mud becomes mudstone or shale, and the animal skeletons and plant pieces can become fossils.
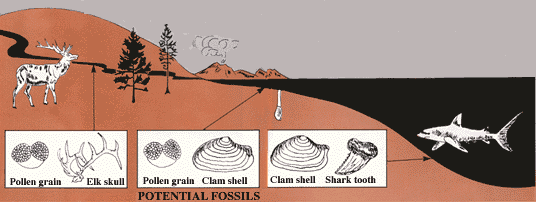
Figure 6. An idealized view of a modern landscape and some of the plants and animals that could be preserved as fossils.
Figure 7. Origins of Major Life Forms
As early as the mid-1600’s, the Danish scientist Nicholas Steno studied the relative positions of sedimentary rocks. He found that solid particles settle from a fluid according to their relative weight or size. The largest, or heaviest, settle first, and the smallest, or lightest, settle last. Slight changes in particle size or composition result in the formation of layers, also called beds, in the rock. Layering, or bedding, is the most obvious feature of sedimentary rocks.
Sedimentary rocks are formed particle by particle and bed by bed, and the layers are piled one on top of the other. Thus, in any sequence of layered rocks, a given bed must be older than any bed on top of it. This Law of Superposition is fundamental to the interpretation of Earth history, because at any one location it indicates the relative ages of rock layers and the fossils in them. Layered rocks form when particles settle from water or air. Steno’s Law of Original Horizontality states that most sediments, when originally formed, were laid down horizontally. However, many layered rocks are no longer horizontal. Because of the Law of Original Horizontality, we know that sedimentary rocks that are not horizontal either were formed in special ways or, more often, were moved from their horizontal position by later events, such as tilting during episodes of mountain building.
Rock layers are also called strata (the plural form of the Latin word stratum), and stratigraphy is the science of strata. Stratigraphy deals with all the characteristics of layered rocks; it includes the study of how these rocks relate to time.
FOSSILS AND ROCKS
To tell the age of most layered rocks, scientists study the fossils these rocks contain. Fossils provide important evidence to help determine what happened in Earth history and when it happened. The word fossil makes many people think of dinosaurs. Dinosaurs are now featured in books, movies, and television programs, and the bones of some large dinosaurs are on display in many museums. These reptiles were dominant animals on Earth for well over 100 million years from the Late Triassic through the Late Cretaceous. Many dinosaurs were quite small, but by the middle of the Mesozoic Period, some species weighed as much as 80 tons. By around 65 million years ago all dinosaurs were extinct. The reasons for and the rapidity of their extinction are a matter of intense debate among scientists.
In spite of all of the interest in dinosaurs, they form only a small fraction of the millions of species that live and have lived on Earth. The great bulk of the fossil record is dominated by fossils of animals with shells and microscopic remains of plants and animals, and these remains are widespread in sedimentary rocks. It is these fossils that are studied by most In the late eighteenth and early nineteenth centuries, the English geologist and engineer William Smith and the French paleontologists Georges Cuvier and Alexandre Brongniart discovered that rocks of the same age may contain the same fossils even when the rocks are separated by long distances. They published the first geologic maps of large areas on which rocks containing similar fossils were shown. By careful observation of the rocks and their fossils, these men and other geologists were able to recognize rocks of the same age on opposite sides of the English Channel. William Smith was able to apply his knowledge of fossils in a very practical way. He was an engineer building canals in England, which has lots of vegetation and few surface exposures of rock. He needed to know what rocks he could expect to find on the hills through which he had to build a canal. Often he could tell what kind of rock was likely to be below the surface by examining the fossils that had eroded from the rocks of the hillside or by digging a small hole to find fossils. Knowing what rocks to expect allowed Smith to estimate costs and determine what tools were needed for the job.
Smith and others knew that the succession of life forms preserved as fossils is useful for understanding how and when the rocks formed. Only later did scientists develop a theory to explain that succession.
FOSSIL SUCCESSION
Three concepts are important in the study and use of fossils:
(1) Fossils represent the remains of once-living organisms.
(2) Most fossils are the remains of extinct organisms; that is, they belong to species that are no longer living anywhere on Earth.
(3) The kinds of fossils found in rocks of different ages differ because life on Earth has changed through time.
Figure 11. Stratigraphic ranges and origins of some major groups of animals and plants.
If we begin at the present and examine older and older layers of rock, we will come to a level where no fossils of humans are present. If we continue backwards in time, we will successively come to levels where no fossils of flowering plants are present, no birds, no mammals, no reptiles, no four-footed vertebrates, no land plants, no fishes, no shells, and no animals. The three concepts are summarized in the general principle called the Law of Fossil Succession: The kinds of animals and plants found as fossils change through time. When we find the same kinds of fossils in rocks from different places, we know that the rocks are the same age.
How do scientists explain the changes in life forms, which are obvious in the record of fossils in rocks? Early explanations were built around the idea of successive natural disasters or catastrophes that periodically destroyed life. After each catastrophe, life began anew. In the mid-nineteenth century, both Charles Darwin and Alfred Wallace proposed that older species of life give rise to younger ones. According to Darwin, this change or evolution is caused by four processes: variation, over-reproduction, competition, and survival of those best adapted to the environment in which they live. Darwin’s theory accounts for all of the diversity of life, both living and fossil. His explanation gave scientific meaning to the observed succession of once-living species seen as fossils in the record of Earth’s history preserved in the rocks. Scientific theories are continually being corrected and improved, because theory must always account for known facts and observations. Therefore, as new knowledge is gained, a theory may change. Application of theory allows us to develop new plants that resist disease, to transplant kidneys, to find oil, and to establish the age of our Earth. Darwin’s theory of evolution has been refined and modified continuously as new information has accumulated. All of the new information has supported Darwin’s basic concept—that living beings have changed through time and older species are ancestors of younger ones.
Figure 13. A species is the most basic unit of classification for living things. This group of fossil clams shows likely ancestor-descendant relationships at the species level. These fossils from the Mid-Atlantic States show the way species can change through time. Notice how the shape of the posterior (rear) end of these clams becomes more rounded in the younger species, and the area where the two shells are held together (ligamental cavity) gets larger. Paleontologists pay particular attention to the shape of the shells and the details of the anatomy preserved as markings on the shells.
Numbers in the left-hand column refer to the following geologic time segments:
1, Pliocene;
2, Miocene;
3, Oligocene;
4, Eocene;
5, Paleocene;
6, Late Cretaceous.
The Law of Fossil Succession is very important to geologists who need to know the ages of the rocks they are studying. The fossils present in a rock exposure or in a core hole can be used to determine the ages of rocks very precisely. Detailed studies of many rocks from many places reveal that some fossils have a short, well-known time of existence. These useful fossils are called index fossils.
Today the animals and plants that live in the ocean are very different from those that live on land, and the animals and plants that live in one part of the ocean or on one part of the land are very different from those in other parts. Similarly, fossil animals and plants from different environments are different. It becomes a challenge to recognize rocks of the same age when one rock was deposited on land and another was deposited in the deep ocean. Scientists must study the fossils from a variety of environments to build a complete picture of the animals and plants that were living at a particular time in the past.
The study of fossils and the rocks that contain them occurs both out of doors and in the laboratory. The field work can take place anywhere in the world. In the laboratory, rock saws, dental drills, pneumatic chisels, inorganic and organic acids, and other mechanical and chemical procedures may be used to prepare samples for study. Preparation may take days, weeks, or months—large dinosaurs may take years to prepare. Once the fossils are freed from the rock, they can be studied and interpreted. In addition, the rock itself provides much useful information about the environment in which it and the fossils were formed.
Figure 14. Fossils can be used to recognize rocks of the same or different ages. The fossils in this figure are the remains of microscopic algae. The pictures shown were made with a scanning electron microscope and have been magnified about 250 times. In South Carolina, three species are found in a core of rock. In Virginia, only two of the species are found. We know from the species that do occur that the rock record from the early part of the middle Eocene is missing in Virginia. We also use these species to recognize rocks of the same ages (early Eocene and latter part of the middle Eocene) in both South Carolina and Virginia. The study of layered rocks and the fossils they contain is called biostratigraphy; the prefix bio is Greek and means life.
THE NUMERIC TIME SCALE
Figure 15. Geologic time scale showing both relative and numeric ages. Ages in millions of years are approximate
Thus far we have been discussing the relative time scale. How can we add numbers to our time
scale? How have geologists determined that:
. Earth is about 4.6 billion years old?
. The oldest known fossils are from rocks that were deposited about 3.5 billion years ago?
. The first abundant shelly fossils occur in rocks that are about 570 million years old?
. The last ice age ended about 10,000 ago?
Nineteenth-century geologists and paleontologists believed that Earth was quite old, but they had only crude ways of estimating just how old. The assignment of ages of rocks in thousands, millions, and billions of years was made possible by the discovery of radioactivity. Now we can use minerals that contain naturally occurring radioactive elements to calculate the numeric age of a rock in years.
The basic unit of each chemical element is the atom. An atom consists of a central nucleus, which contains protons and neutrons, surrounded by a cloud of electrons. Isotopes of an element are atoms that differ from one another only in the number of neutrons in the nucleus. For example, radioactive atoms of the element potassium have 19 protons and 21 neutrons in the nucleus (potassium 40); other atoms of potassium have 19 protons and 20 or 22 neutrons (potassium 39 and potassium 41). A radioactive isotope (the parent) of one chemical element naturally converts to a stable isotope (the daughter) of another chemical element by undergoing changes in the nucleus.
The change from parent to daughter happens at a constant rate, called the half-life. The half-life of a radioactive isotope is the length of time required for exactly one-half of the parent atoms to decay to daughter atoms. Each radioactive isotope has its own unique half-life. Precise laboratory measurements of the number of remaining atoms of the parent and the number of atoms of the new daughter produced are used to compute the age of the rock. For dating geologic materials, four parent/daughter decay series are especially useful: carbon to nitrogen, potassium to argon, rubidium to strontium, and uranium to lead. Age determinations using radioactive isotopes are subject to relatively small errors in measurement—but errors that look small can mean many years or millions of years. If the measurements have an error of 1 percent, for example, an age determination of 100 million years could actually be wrong by a million years too low or too high.
Isotopic techniques are used to measure the time at which a particular mineral within a rock was formed. To allow us to assign numeric ages to the geologic time scale, a rock that can be dated isotopically is found together with rocks that can be assigned relative ages because of their fossils. Many samples, usually from several different places, must be studied before assigning a numeric age to a boundary on the geologic time scale.
Figure 16. Parents and daughters for some isotopes commonly used to establish numeric ages of rocks.
The geologic time scale is the product of many years of detective work, as well as a variety of dating techniques not discussed here. The details will change as more and better information and tools become available. Many scientists have contributed and continue to contribute to the refinement of the geologic time scale as they study the fossils and the rocks, and the chemical and physical properties of the materials of which Earth is made. Just as in the time of William Smith, knowing what kinds of rocks are found below the soil can help people to make informed judgments about the uses of the resources of the planet.